Research
Interests
NMR-based Computational Structural Biology
Computation methods to study biomolecular systems, in particular by nuclear magnetic resonance (NMR), are the focus of our research. The relationship between structure, dynamics and function of biological macromolecules is of fundamental importance for understanding life at a molecular level, and a key element of rational drug design. The three-dimensional structure has a pivotal role, since its knowledge is essential to understand the physical, chemical, and biological properties of a protein. Until recently NMR protein structure determination was a laborious undertaking that occupied a trained spectroscopist for months or years for each new protein structure. This situation has changed by the introduction of automated, computational systems. We are extending NMR protein structure analysis to hitherto inaccessible systems, including proteins larger than 40 kDa, membrane proteins, and proteins studied directly inside living cells.
- Protein structure analysis
- Protein structure analysis
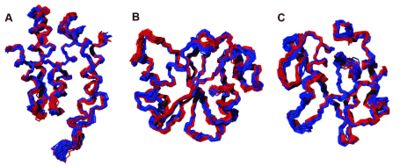
- Three-dimensional structures of proteins in solution can be calculated on the basis of conformational restraints derived from NMR measurements. Our CYANA program package, based on simulated annealing by molecular dynamics simulation in torsion angle space and the automated assignment of NOE distance restraints, is one of the most widely used algorithms for this purpose. Automated methods for protein structure determination by NMR have increasingly gained acceptance and are now widely used for the automated assignment of distance restraints and the calculation of three-dimensional structures. Our FLYA algorithm for the fully automated NMR structure determination of proteins is suitable to substitute all manual spectra analysis and thus overcomes a major efficiency limitation of the NMR method for protein structure determination. Fully automated structure determination of proteins in solution (FLYA) yields, without human intervention, three-dimensional protein structures starting from a set of multidimensional NMR spectra. As in the classical manual approach, structures are determined by a set of experimental NOE distance restraints without reference to already existing structures or empirical molecular modeling information. In addition to the three-dimensional structure of the protein, FLYA yields backbone and side-chain chemical shift assignments, and cross peak assignments for all spectra.
- [3] Koglin, A., Löhr, F., Bernhard, F., Rogov, V. V., Frueh, D. P., Strieter, E. R., Mofid, M. R., Güntert, P., Wagner, G., Walsh, C. T., Marahiel, M. A. & Dötsch, V. (2008). Structural basis for the selectivity of the external thioesterase of the surfactin synthetase. Nature 454, 907-911.
- [4] Güntert, P. (2009). Automated structure determination from NMR spectra. Eur. Biophys. J. 38, 129-143.
- [5] López-Méndez, B., & Güntert, P. (2006). Automated protein structure determination from NMR spectra. J. Am. Chem. Soc. 128, 13112–13122.
- Stereo-array isotope labeling
- Stereo-array isotope labeling
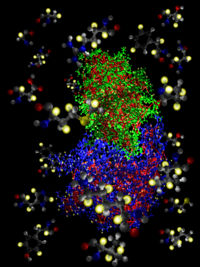
- NMR spectroscopy can determine the three-dimensional structure of proteins in solution. Nevertheless, its potential has been limited by the difficulty of interpreting NMR spectra in the presence of broadened and overlapped resonance lines and low signal-to-noise ratios. Stereo-array isotope labelling (SAIL) can overcome many of these problems by applying a complete stereo- and regiospecific pattern of stable isotopes, which is optimal with regard to the quality and information content of the resulting NMR spectra. SAIL utilizes exclusively chemically and enzymatically synthesized amino acids for cell-free protein expression such that in all methylene groups, one 1H is stereo-selectively replaced by 2H, in all single methyl groups, two 1H are replaced by 2H, and in the prochiral methyl groups of Leu and Val, one methyl is stereo-selectively -12C(2H)3 and the other is -13C1H(2H)2. In six-membered aromatic rings, the 12C-2H and 13C-1H moieties alternate with each other. SAIL achieves a 4–7-fold increase in signal-to-noise, sharper resonance lines, and a 40–60% reduction in the number of signals without sacrificing essential information about the backbones and the side chains of all amino acid residue types. The resulting reduction of spectral overlap, the higher accuracy of the frequency determination, the complete stereospecific assignment, and the measurement of longer 1H–1H distances makes it possible to determine high-quality solution structures of proteins larger than 30 kDa.
- Kainosho, M., Torizawa, T., Iwashita, Y., Terauchi, T., Ono, A. M. & Güntert, P. Optimal isotope labeling for NMR protein structure determinations. Nature 440, 52–57 (2006)
- Protein structure determination in living cells by in-cell NMR spectroscopy
- Protein structure determination in living cells by in-cell NMR spectroscopy
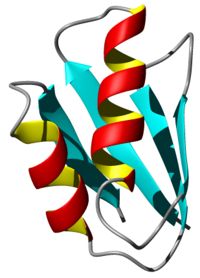
- Proteins in living cells work in an extremely crowded environment where they interact specifically with other proteins, nucleic acids, co-factors and ligands. Methods for the three-dimensional structure determination of purified proteins in single crystals or in solution are widely used and have made very valuable contributions to understanding many biological processes. However, replicating the cellular environment in vitro is difficult. In vivo observations of three-dimensional structures, dynamics and interactions of proteins are required for fully understanding the structural basis of their functions inside cells. Investigating proteins “at work” in a living environment at atomic resolution is thus a major goal of molecular biology. Recent developments in NMR hardware and methodology have enabled the measurement of high-resolution heteronuclear multi-dimensional NMR spectra of macromolecules in living cells (in-cell NMR). Various intracellular events such as conformational changes, dynamics and binding events have been investigated by this method. However, the low sensitivity and short life time of the samples have so far prevented the acquisition of sufficient structural information to determine protein structures by in-cell NMR. Recently we presented the first three-dimensional protein structure calculated exclusively on the basis of information obtained in living cells. The in-cell NMR approach can thus provide accurate high-resolution structures of proteins in living environments.
- Sakakibara, D., Sasaki, A., Ikeya, T., Hamatsu, J., Hanashima, T., Mishima, M., Yoshimasu, M., Hayashi, N., Mikawa, T., Wälchli, M., Smith, B. O., Shirakawa, M., Güntert, P. & Ito, Y. Protein structure determination in living cells by in-cell NMR spectroscopy. Nature 458, 102-105 (2009)